Voltammetry
Last Updated: 1/5/23 by Alex Peroff
Article Contents/Section Navigation
-
General Overview
-
References
1General Overview
The term voltammetry refers broadly to any method where the electrode potential is varied while the current is measured.
The terminology associated with voltammetry varies across different industries and academic disciplines, but the underlying principles of all voltammetric techniques are very similar.
The most common form of voltammetry involves sweeping the electrode potential from an initial value to a final value at a constant rate. When working in the context of electroanalytical chemistry with a non-rotating electrode, this technique is called linear sweep voltammetry (LSV).
In the context of corrosion science, this kind of technique is usually called linear polarization resistance (LPR) or a Tafel analysis. The term cyclic voltammetry (CV) refers to a method where the electrode potential is swept repeatedly back and forth between two extremes.
Commonly, the working electrode is kept still in solution as to limit convective mass transport of electrochemically active species to the electrode surface (relying predominantly on diffusion); however, additional applications of voltammetry change the mass transport limitation to extend beyond simple diffusion. Often this is achieved by using a rotating electrode. When working with a rotating electrode, it is common to further specify the kind of electrode being used as part of the technique name, such as rotating disk voltammetry (RDE), rotating ring-disk voltammetry (RRDE), or rotating cylinder voltammetry (RCE). In each of these techniques, the rotation rate is held constant as the electrode is swept from one potential to another potential at a constant sweep rate. In electroanalytical chemistry, the potential sweep usually spans at least 200 mV on either side of the standard electrode potential, and rotation rates are usually between 100 and 2400 RPM. However, in the context of a corrosion study, the potential sweep may span a much narrower range (50 mV) using a slower sweep rate (less than 5 mV/sec) with an emphasis on higher rotation rates.
As an example, consider a solution that initially contains only the oxidized form of a molecule or ion. A rotating electrode is placed in this solution and is initially poised at a potential that is more positive than the standard potential. At this potential, there is little or no current because there is nothing to oxidize (the molecule or ion is already oxidized), and the potential is not negative enough to cause any appreciable reduction of the molecule or ion.
Next, the electrode potential is slowly (20 mV/sec) swept in the negative (cathodic) direction (Fig 1. left). As the applied potential approaches the standard electrode potential, a cathodic current is observed (Fig 1. right). The cathodic current continues to increase as the potential moves past the standard electrode potential towards more negative potentials.
The current eventually reaches a maximum value (limiting current) once the applied potential is sufficiently negative relative to the standard electrode potential. At such a negative potential, any oxidized form of the molecule or ion

that reaches the surface of the electrode is immediately converted to the reduced form (R) as shown below.
 |
(6) |
The observed cathodic current is the result of electrons flowing out of the electrode and into the solution. The rate of electron flow is limited only by how fast the oxidized form

can arrive at the electrode surface. The maximum current observed in this circumstance is called the cathodic limiting current (i
LC).
Whenever an observed current is limited only by the rate at which material arrives at the electrode surface, the current is said to be mass transport limited. When working with a rotating electrode, the rate of mass transport is related to the rotation rate of the electrode. Rotating the electrode at a faster rate increases the rate at which material arrives at the electrode surface. Thus, the limiting current increases with increasing rotation rate. Experiments involving a rotating electrode are designed to purposefully exploit this fundamental relationship between the rotation rate and the limiting current.
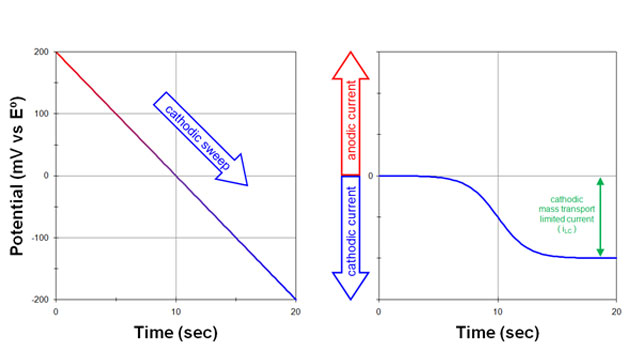
Fig 1. Response to a potential sweep (cathodic) from a solution initially containing only the oxidized form (O) with no reduced form (R)
The cathodic sweep experiment described above (Fig 1) applies to the case where the solution initially contains only the oxidized form O of the molecule or ion being studied. The opposite case yields similar results. Consider a solution that initially contains only the reduced form R of the molecule or ion being studied. The rotating electrode is initially poised at a potential that is about 200 mV more negative than the standard potential. At this potential, there is little or no current because there is nothing to reduce (the molecule or ion is already reduced), and the potential is not positive enough to cause any appreciable oxidation of the molecule or ion.
Next, the electrode potential is slowly swept in the positive (anodic) direction (Fig 2. left) and an anodic current is observed (Fig 2. right). The anodic current eventually reaches a maximum value when the potential is sufficiently positive relative to the standard electrode potential. At this point, any of the reduced form (R) that reaches the electrode surface is immediately converted to the oxidized form (O).
 |
(7) |
The observed current is the result of electrons flowing into the electrode. The maximum current observed is called the anodic limiting current (iLA).
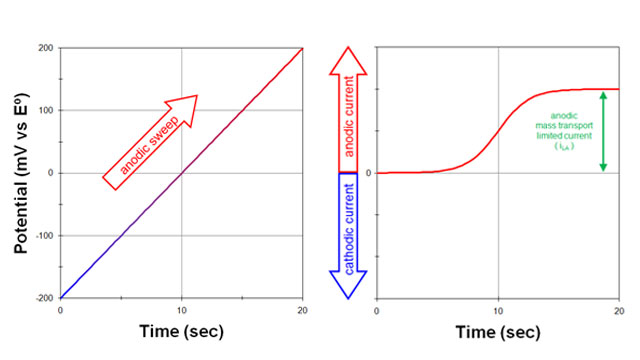
Fig 2. Response to a potential sweep (anodic) from a solution initially containing only the reduced form (R) with no oxidized form (O)
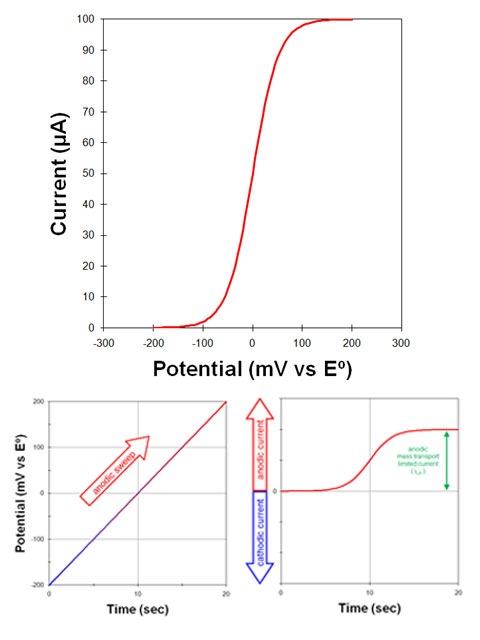
Fig 3. A voltammogram is a plot of current versus potential
2References