Probing Fuel Cell Electrocatalysts with RDE and RRDE
Last Updated: 1/5/23 by Alex Peroff
Download as PDF1Introduction


Albery, W. J.; Hitchman, M. L.  Ring-Disc Electrodes Oxford University Press: Oxford, England, 1971.
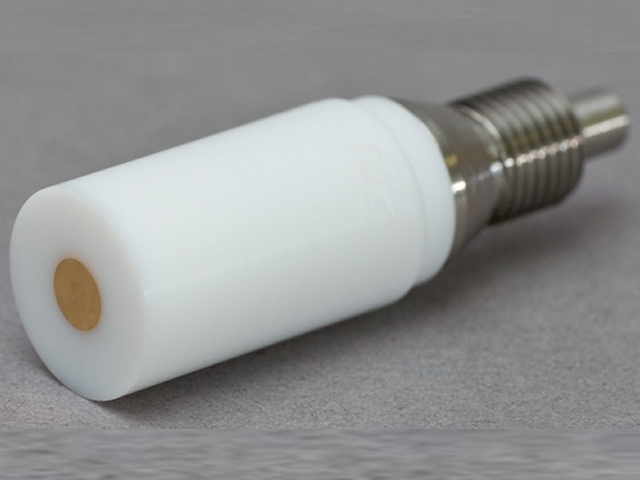
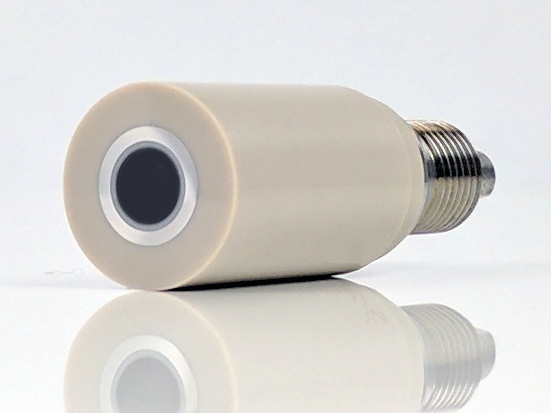
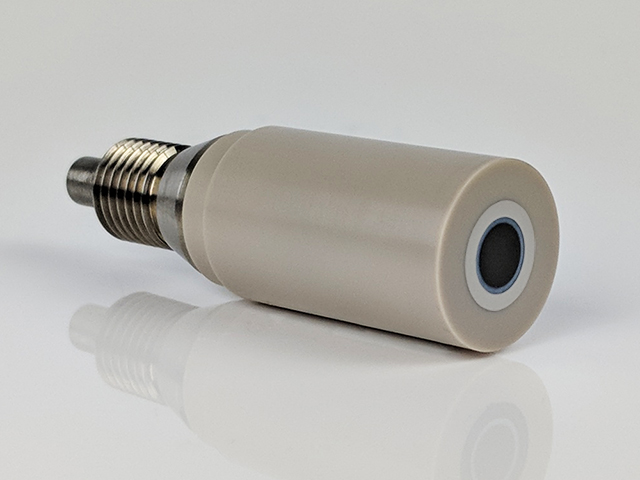
Figure 1. E8 Series RRDE Tip, Glassy Carbon Disk, Platinum Ring
2Electrode Rotation
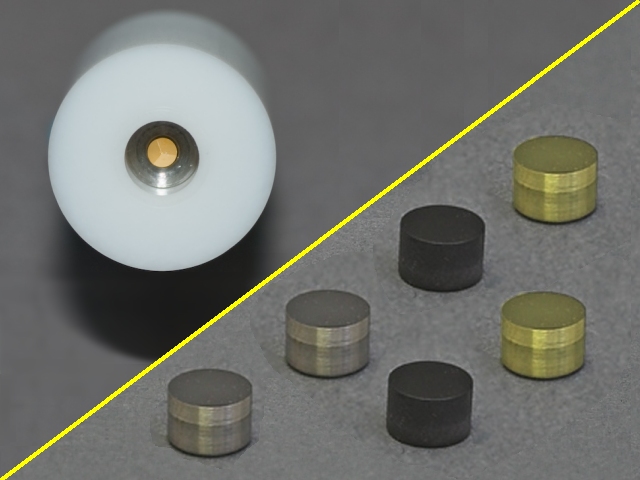
Figure 2. E5TQ Series ChangeDisk RDE Tip in PTFE
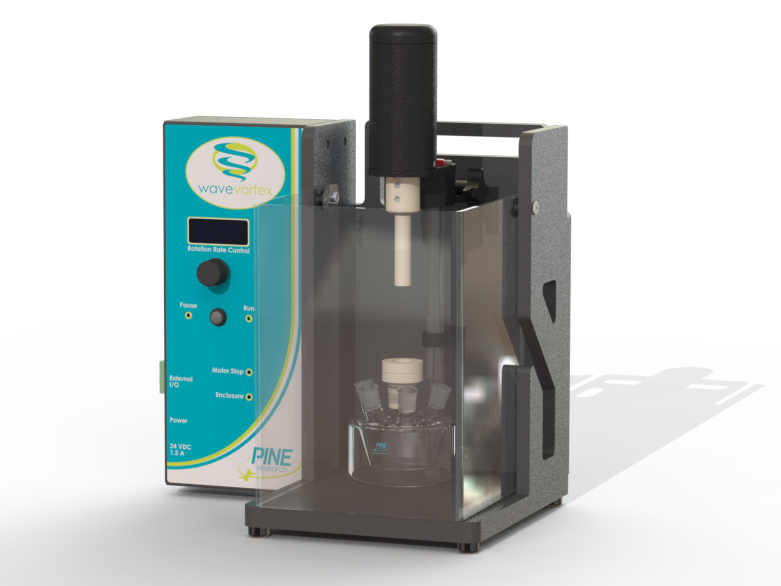
Figure 3. WaveVortex 10 Electrode Rotator
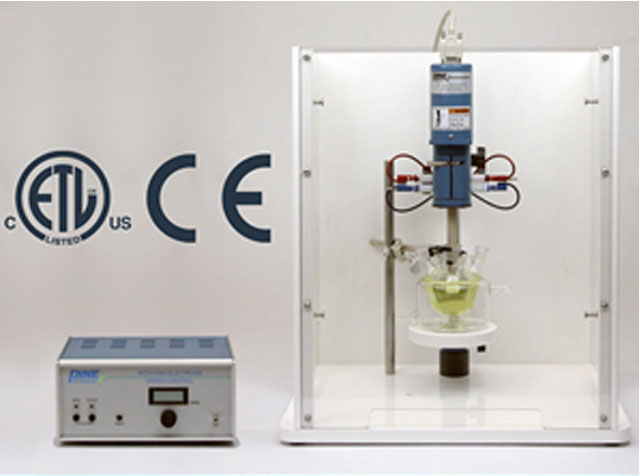
Figure 4. Pine Research Modulated Speed Rotator (MSR)
3Basic Concepts

3.1RDE Kinetic Measurements

Paulus, U. A.; Wokaun, A.; Scherer, G. G.; Schmidt, T. J.; Stamenkovic, V.; Markovic, N. M.; Ross, P. N.  Oxygen reduction on high surface area Pt-based alloy catalysts in comparison to well defined smooth bulk alloy electrodes.  Electrochim. Acta, 2002, 47(22-23), 3787–3798.
Paulus, U. A.; Schmidt, T. J.; Gasteiger, H. A.; Behm, R. J.  Oxygen reduction on a high-surface area Pt/Vulcan carbon catalyst: A thin-film rotating ring-disk electrode study.  J. Electroanal. Chem., 2001, 495(2), 134–145.
Schmidt, T. J.; Paulus, U. A.; Gasteiger, H. A.; Behm, R. J.  The oxygen reduction reaction on a Pt/carbon fuel cell catalyst in the presence of chloride anions.  J. Electroanal. Chem., 2001, 508(1-2), 41–47. By gradually increasing the rotation rate of the RDE, the rate of mass transport of the dissolved oxygen to the electrode surface increases. Initially, the cathodic (reduction) current at the disk electrode is governed by this mass transport (i.e., the rate at which oxygen is arriving at the electrode). As the rotation rate increases, the current increases as the amount of oxygen arriving at the electrode surface increases.

Albery, W. J.; Hitchman, M. L.  Ring-Disc Electrodes Oxford University Press: Oxford, England, 1971.
3.2RRDE Peroxide Detection

Albery, W. J.; Hitchman, M. L.  Ring-Disc Electrodes Oxford University Press: Oxford, England, 1971.
Gasteiger, H. A.; Kocha, S. S.; Sompalli, B.; Wagner, F. T.  Activity benchmarks and requirements for Pt, Pt-alloy, and non-Pt oxygen reduction catalysts for PEMFCs.  Appl. Catal., B, 2005, 56(1-2 SPEC. ISS.), 9–35.
Paulus, U. A.; Wokaun, A.; Scherer, G. G.; Schmidt, T. J.; Stamenkovic, V.; Markovic, N. M.; Ross, P. N.  Oxygen reduction on high surface area Pt-based alloy catalysts in comparison to well defined smooth bulk alloy electrodes.  Electrochim. Acta, 2002, 47(22-23), 3787–3798.
Paulus, U. A.; Schmidt, T. J.; Gasteiger, H. A.; Behm, R. J.  Oxygen reduction on a high-surface area Pt/Vulcan carbon catalyst: A thin-film rotating ring-disk electrode study.  J. Electroanal. Chem., 2001, 495(2), 134–145.
Schmidt, T. J.; Paulus, U. A.; Gasteiger, H. A.; Behm, R. J.  The oxygen reduction reaction on a Pt/carbon fuel cell catalyst in the presence of chloride anions.  J. Electroanal. Chem., 2001, 508(1-2), 41–47.

4Practical Matters

4.1Polishing Electrodes

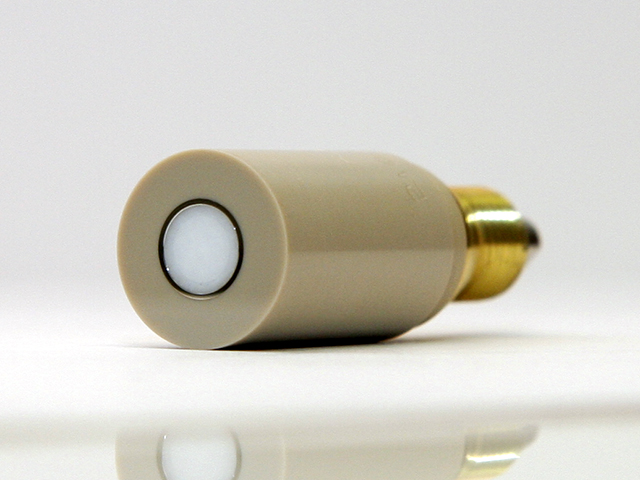
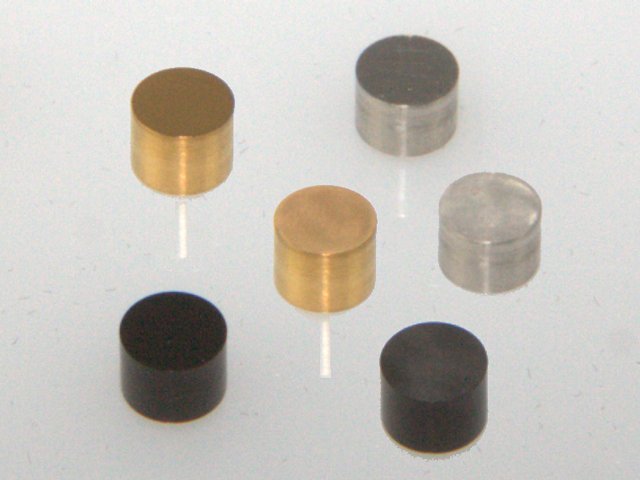
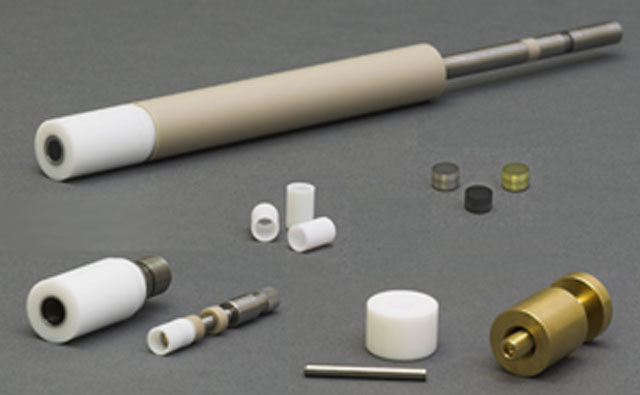
Figure 5. Some Rotating Ring-Disk Electrode (RRDE) Designs may be Completely Disassembled to Facilitate Polishing, Catalyst Preparation, and Subsequent Imaging of Disk Surface
4.2Coating Catalyst on Disk Electrode
4.3Rotation Rates
4.4Collection Efficiency

Theoretical Determination of Collection Efficiency (N) not all the product generated at the disk electrode will make the trip to the ring electrode. The flow pattern at a rotating electrode generally sweeps anywhere from 20% to 30% of the disk products past the ring electrode. The percentage of material which is collected (detected) at the ring electrode is often called the collection efficiency of the RRDE.



4.5Elevated Temperatures
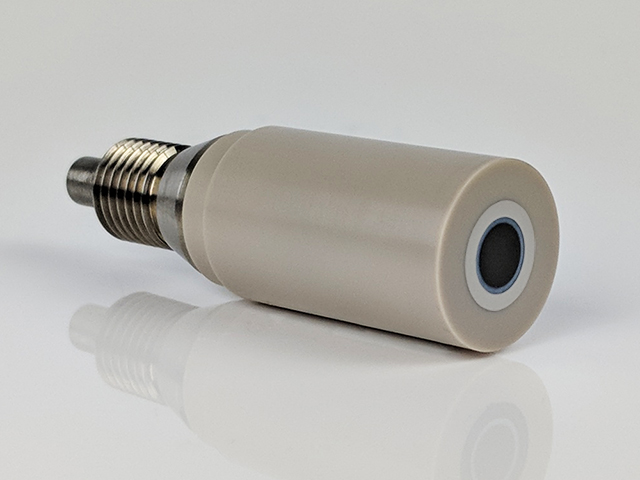

Paulus, U. A.; Wokaun, A.; Scherer, G. G.; Schmidt, T. J.; Stamenkovic, V.; Markovic, N. M.; Ross, P. N.  Oxygen reduction on high surface area Pt-based alloy catalysts in comparison to well defined smooth bulk alloy electrodes.  Electrochim. Acta, 2002, 47(22-23), 3787–3798.
Paulus, U. A.; Schmidt, T. J.; Gasteiger, H. A.; Behm, R. J.  Oxygen reduction on a high-surface area Pt/Vulcan carbon catalyst: A thin-film rotating ring-disk electrode study.  J. Electroanal. Chem., 2001, 495(2), 134–145.
Schmidt, T. J.; Paulus, U. A.; Gasteiger, H. A.; Behm, R. J.  The oxygen reduction reaction on a Pt/carbon fuel cell catalyst in the presence of chloride anions.  J. Electroanal. Chem., 2001, 508(1-2), 41–47. PTFE-shrouded RRDEs are easily damaged by such exposure to high temperatures. Sometimes this damage (usually a leak) can be repaired by replacing the PTFE spacer between the disk and the ring electrode. This piece is called the PTFE U-Cup,
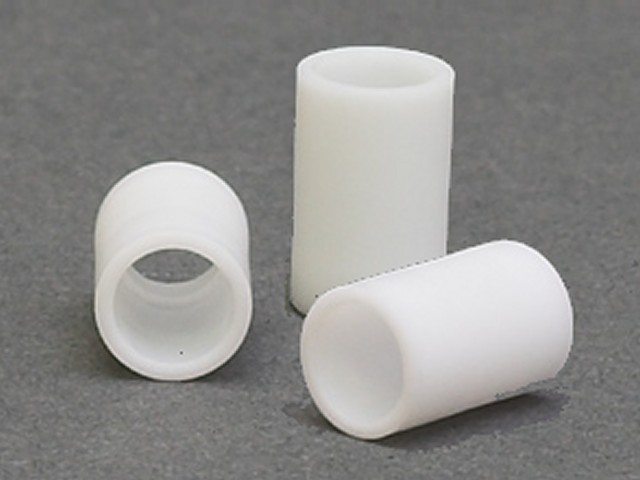
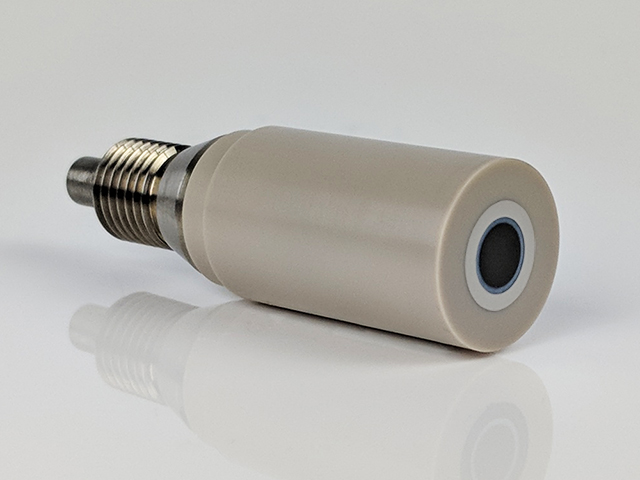
5Additional Reading

Geniès, L.; Faure, R.; Durand, R.  Electrochemical reduction of oxygen on platinum nanoparticles in alkaline media.  Electrochim. Acta, 1998, 44(8-9), 1317–1327.
Higuchi, E.; Uchida, H.; Watanabe, M.  Effect of loading level in platinum-dispersed carbon black electrocatalysts on oxygen reduction activity evaluated by rotating disk electrode.  J. Electroanal. Chem., 2005, 583(1), 69–76.
Wei, Z. D.; Chan, S. H.; Li, L. L.; Cai, H. F.; Xia, Z. T.; Sun, C. X.  Electrodepositing Pt on a Nafion-bonded carbon electrode as a catalyzed electrode for oxygen reduction reaction.  Electrochim. Acta, 2005, 50(11), 2279–2287.
Marcotte, S.; Villers, D.; Guillet, N.; Roué, L.; Dodelet, J. P.  Electroreduction of oxygen on Co-based catalysts: Determination of the parameters affecting the two-electron transfer reaction in an acid medium.  Electrochim. Acta, 2004, 50(1), 179–188.
Durón, S.; Rivera-Noriega, R.; Nkeng, P.; Poillerat, G.; Solorza-Feria, O.  Kinetic study of oxygen reduction on nanoparticles of ruthenium synthesized by pyrolysis of Ru3(CO)12.  J. Electroanal. Chem., 2004, 566(2), 281–289.
El-Deab, M. S. ; Ohsaka, T.   Electrocatalysis by nanoparticles: oxygen reduction on gold nanoparticles-electrodeposited platinum electrodes.  J. Electroanal. Chem., 2003, 553, 107-115.
Guo, F.; Yang, H.; Aguila, B.; Al-Enizi, A. M.; Nafady, A.; Singh, M.; Bansal, V.; Ma, S.  Cobalt nanoparticles incorporated into hollow doped porous carbon capsules as a highly efficient oxygen reduction electrocatalyst.  Catal. Sci. Technol., 2018, 8(20), 5244-5250.
Jiang, S. ; Ithisuphalap, K. ; Zeng, X. ; Wu, G. ; Yang, H.   3D porous cellular NiCoO2/graphene network as a durable bifunctional electrocatalyst for oxygen evolution and reduction reactions.  J. Power Sources, 2018, 399, 66-75.
Boone, C. V. ; Maia, G.   Lowering metal loadings onto Pt–Pd–Cu/graphene nanoribbon nanocomposites affects electrode collection efficiency and oxygen reduction reaction performance.  Electrochim. Acta, 2019, 303, 192-203.
Gao, R. ; Yin, Y. ; Niu, F. ; Wang, A. ; Li, S. ; Dong, H. ; Yang, S.   One Pot Synthesis of FeCo/N-Doped 3D Porous Carbon Nanosheets as Bifunctional Electrocatalyst for the Oxygen Reduction and Evolution Reactions.  ChemElectroChem, 2019.
He, X. ; Yi, X. ; Yin, F. ; Chen, B. ; Li, G. ; Yin, H.   Less active CeO2 regulating bifunctional oxygen electrocatalytic activity of Co3O4@N-doped carbon for Zn–air batteries.  J. Mater. Chem. A, 2019.
Kaviani, S. ; Mohammadi Ghaleni, M. ; Tavakoli, E. ; Nejati, S.   Electroactive and Conformal Coatings of Oxidative Chemical Vapor Deposition Polymers for Oxygen Electroreduction.  ACS Appl. Polym. Mater., 2019.
Lenarda, A. ; Bevilacqua, M. ; Tavagnacco, C. ; Nasi, L. ; Criado, A. ; Vizza, F. ; Melchionna, M. ; Prato, M. ; Fornasiero, P.   Selective H2O2 electrocatalytic generation by Cobalt@N-doped graphitic carbon core-shell nanohybrids.  ChemSusChem, 2019.
Madkikar, P. ; Menga, D. ; Harzer, G. S. ; Mittermeier, T. ; Siebel, A. ; Wagner, F. E. ; Merz, M. ; Schuppler, S. ; Nagel, P. ; Muñoz-García, A. B. ; Pavone, M. ; Gasteiger, H. A. ; Piana, M.   Nanometric Fe-Substituted ZrO2 on Carbon Black as PGM-Free ORR Catalyst for PEMFCs.  Journal of The Electrochemical Society, 2019, 166(7), F3032-F3043.
Yu, Q. ; Yin, S. ; Zhang, J. ; Yin, H.   Structure dependent activity and durability towards oxygen reduction reaction on Pt modified nanoporous gold.  Electrochim. Acta, 2019, 298, 599-608.
6References
- Ball, S. C.  Electrochemistry of Proton Conducting Membrane Fuel Cells.  Platinum Met. Rev., 2005, 49(1), 27-32.
- Bard, A. J.; Faulkner, L. A.  Electrochemical Methods: Fundamentals and Applications, 2nd ed. Wiley-Interscience: New York, 2000.
- Albery, W. J.; Hitchman, M. L.  Ring-Disc Electrodes Oxford University Press: Oxford, England, 1971.
- Gasteiger, H. A.; Kocha, S. S.; Sompalli, B.; Wagner, F. T.  Activity benchmarks and requirements for Pt, Pt-alloy, and non-Pt oxygen reduction catalysts for PEMFCs.  Appl. Catal., B, 2005, 56(1-2 SPEC. ISS.), 9–35.
- Paulus, U. A.; Wokaun, A.; Scherer, G. G.; Schmidt, T. J.; Stamenkovic, V.; Markovic, N. M.; Ross, P. N.  Oxygen reduction on high surface area Pt-based alloy catalysts in comparison to well defined smooth bulk alloy electrodes.  Electrochim. Acta, 2002, 47(22-23), 3787–3798.
- Paulus, U. A.; Schmidt, T. J.; Gasteiger, H. A.; Behm, R. J.  Oxygen reduction on a high-surface area Pt/Vulcan carbon catalyst: A thin-film rotating ring-disk electrode study.  J. Electroanal. Chem., 2001, 495(2), 134–145.
- Schmidt, T. J.; Paulus, U. A.; Gasteiger, H. A.; Behm, R. J.  The oxygen reduction reaction on a Pt/carbon fuel cell catalyst in the presence of chloride anions.  J. Electroanal. Chem., 2001, 508(1-2), 41–47.
- Brisard, G.; Bertrand, N.; Ross, P. N.; Marković, N. M.  Oxygen reduction and hydrogen evolution–oxidation reactions on Cu(hkl) surfaces.  J. Electroanal. Chem., 2000, 480(1-2), 219–224.
- Geniès, L.; Faure, R.; Durand, R.  Electrochemical reduction of oxygen on platinum nanoparticles in alkaline media.  Electrochim. Acta, 1998, 44(8-9), 1317–1327.
- Higuchi, E.; Uchida, H.; Watanabe, M.  Effect of loading level in platinum-dispersed carbon black electrocatalysts on oxygen reduction activity evaluated by rotating disk electrode.  J. Electroanal. Chem., 2005, 583(1), 69–76.
- Wei, Z. D.; Chan, S. H.; Li, L. L.; Cai, H. F.; Xia, Z. T.; Sun, C. X.  Electrodepositing Pt on a Nafion-bonded carbon electrode as a catalyzed electrode for oxygen reduction reaction.  Electrochim. Acta, 2005, 50(11), 2279–2287.
- Marcotte, S.; Villers, D.; Guillet, N.; Roué, L.; Dodelet, J. P.  Electroreduction of oxygen on Co-based catalysts: Determination of the parameters affecting the two-electron transfer reaction in an acid medium.  Electrochim. Acta, 2004, 50(1), 179–188.
- Durón, S.; Rivera-Noriega, R.; Nkeng, P.; Poillerat, G.; Solorza-Feria, O.  Kinetic study of oxygen reduction on nanoparticles of ruthenium synthesized by pyrolysis of Ru3(CO)12.  J. Electroanal. Chem., 2004, 566(2), 281–289.
- El-Deab, M. S. ; Ohsaka, T.   Electrocatalysis by nanoparticles: oxygen reduction on gold nanoparticles-electrodeposited platinum electrodes.  J. Electroanal. Chem., 2003, 553, 107-115.
- Guo, F.; Yang, H.; Aguila, B.; Al-Enizi, A. M.; Nafady, A.; Singh, M.; Bansal, V.; Ma, S.  Cobalt nanoparticles incorporated into hollow doped porous carbon capsules as a highly efficient oxygen reduction electrocatalyst.  Catal. Sci. Technol., 2018, 8(20), 5244-5250.
- Jiang, S. ; Ithisuphalap, K. ; Zeng, X. ; Wu, G. ; Yang, H.   3D porous cellular NiCoO2/graphene network as a durable bifunctional electrocatalyst for oxygen evolution and reduction reactions.  J. Power Sources, 2018, 399, 66-75.
- Boone, C. V. ; Maia, G.   Lowering metal loadings onto Pt–Pd–Cu/graphene nanoribbon nanocomposites affects electrode collection efficiency and oxygen reduction reaction performance.  Electrochim. Acta, 2019, 303, 192-203.
- Gao, R. ; Yin, Y. ; Niu, F. ; Wang, A. ; Li, S. ; Dong, H. ; Yang, S.   One Pot Synthesis of FeCo/N-Doped 3D Porous Carbon Nanosheets as Bifunctional Electrocatalyst for the Oxygen Reduction and Evolution Reactions.  ChemElectroChem, 2019.
- He, X. ; Yi, X. ; Yin, F. ; Chen, B. ; Li, G. ; Yin, H.   Less active CeO2 regulating bifunctional oxygen electrocatalytic activity of Co3O4@N-doped carbon for Zn–air batteries.  J. Mater. Chem. A, 2019.
- Kaviani, S. ; Mohammadi Ghaleni, M. ; Tavakoli, E. ; Nejati, S.   Electroactive and Conformal Coatings of Oxidative Chemical Vapor Deposition Polymers for Oxygen Electroreduction.  ACS Appl. Polym. Mater., 2019.
- Lenarda, A. ; Bevilacqua, M. ; Tavagnacco, C. ; Nasi, L. ; Criado, A. ; Vizza, F. ; Melchionna, M. ; Prato, M. ; Fornasiero, P.   Selective H2O2 electrocatalytic generation by Cobalt@N-doped graphitic carbon core-shell nanohybrids.  ChemSusChem, 2019.
- Madkikar, P. ; Menga, D. ; Harzer, G. S. ; Mittermeier, T. ; Siebel, A. ; Wagner, F. E. ; Merz, M. ; Schuppler, S. ; Nagel, P. ; Muñoz-García, A. B. ; Pavone, M. ; Gasteiger, H. A. ; Piana, M.   Nanometric Fe-Substituted ZrO2 on Carbon Black as PGM-Free ORR Catalyst for PEMFCs.  Journal of The Electrochemical Society, 2019, 166(7), F3032-F3043.
- Yu, Q. ; Yin, S. ; Zhang, J. ; Yin, H.   Structure dependent activity and durability towards oxygen reduction reaction on Pt modified nanoporous gold.  Electrochim. Acta, 2019, 298, 599-608.